The
Report :"The Production and Properties of Metal-Carbon Composite
Coatings with
Nano-Crystal Structure written High Deposition Rate
Magnetron (HDRM) "
Introduction
The
application of three-coated fuel particles (TCFP) with multi film
preserving coating of PyC and SiC, where SiC both secures the mechanical
strength of TCFP and prevents the diffusion of disintegration metal
products, in particular, Pd, is the basic concept of high temperature
gas-cooled reactor (HTGCR) (pls, see Fig. 1)[1].
However, under the increase of the power density and efficiency of TCFP
service the latter undergoes very high temperature > 1700оС
which makes it problematic for SiC mechanical and chemical resistance to
preserve. Therefore ZrC is considered as a replacement for SiC.
According to [2] ZrC in TCFP withstands both high temperature and more
chemically aggressive Pd and other disintegration products better than
SiC. Thus, more high temperature resistance of ZrC provides better fuel
burning and consequently improving HTGCR economics.
Deposition of MeC
coating
There are two basic
processes of SiC/MeC coatings for TCFP for application in HTGCR:
(i)
chemical vacuum deposition (CVD) utilizing thermal disintegrated
chemical compositions: chlorides, iodides, bromides [3]; the technology
is rather simple but ecologically harmful and, in some cases, explosive,
requires high temperature 450-1600оС to obtain required coatings;
(ii)
vacuum physical evaporation or sputtering materials (PVD)[4]; the
technology is ecologically sound, non-explosive and does not require
high temperature. Among the PVD technologies of depositing chemical
compounds, i.a. carbides, the ionic-plasma magnetron sputtering is more
effective and technological (pls, see Fig. 2).
As a result of long
intensive experience we succeeded in creating the system of high
deposition rate magnetrons (HDRM) with balanced magnetic field and
direct cooling of targets. Such HDRMs make it possible to have the
power density of discharge up to and > 103 W/сm2 and the deposition rate
up to tens mkm per hour. HDRMs are universal and used for depositing
coatings of metals, alloys and chemical compounds (carbides, nitrides,
carbonitrides, etc; pls, see Fig. 3). The basic particularity of HDRM is
high power density of discharge and, as a consequence, a high plasma
concentration in the magnetic catching zone as well as intensive target
erosion. Furthermore, under certain conditions the sputtering rate of
materials in this zone becomes equal [5].
The deposition rate
equalization for different materials occurs under the following
conditions:
-
The magnetic field
under target is symmetric (balanced);
-
The residual
induction of magnetic field under target, B>300 Gs;
-
The power density of
discharge in the magnetic catching zone, P> 20 W/см2;
-
The target erosion
zone is of mosaic structure.
The time for getting
deposition rate equalization depends upon the difference in materials
deposition rate and sputtering coefficients. In practice, for the
majority of materials, when the difference in materials sputtering
coefficients is 2-3 times, the time for deposition rate equalization is
10-15 minutes (the sputtering coefficients of С=0,2 and Zr=0,7 under
U=600V). Вased on the above the simplest and ecologically sound
technology for getting carbides coatings has been proposed. The
technology implies the joint sputtering from one target composed of
metal and carbon where the target components concentration in atomic %
links with their areas correlation in the erosion zone.
The scheme and general
view of such mosaic target is given in Fig. 4.
To get the ZrC coatings
the two types of mosaic targets have been made:
-
50Zr : 50C at.% - to
get under the Zr-C diagram the ZrC0,9-0,95 composition the target
matrix is C where Zr inserts are put into C-matrix;
-
80Zr : 20C аt% - to
get under the Zr-C diagram the ZrC 0,5-0,6 composition the target
matrix is Zr where C inserts are put into Zr-matrix;
The sputtering of the
mosaic targets MeC is carried out in the power density range 41,7 W/см2
– 125,2 W/см2 under the Argon pressure of 3-5х10-1 Pa. The max ZrC
deposition rate is ~ 25-30 mkm/hour with 80 mm distance. The temperature
of surface condensing the thin film is 150-200оС. By using the multi
cathode system of mosaic targets the ZrC deposition rate could be in the
range of 120-240 mkm/hour.
For comparison: the max
ZrC deposition rate of the ZrC target (non-mosaic) is ~ 3-5 mkm/hour;
in the atmosphere of chemically active gas, like propane, the ZrC
deposition rate is 5-7 mkm/hour.
The
composition and structure of MeC coating.
The roentgenographic
investigation of composition and structure of MeC coating shows that
the sputtering of mosaic target leads to the homogeneous mixing of the
atoms of C and Me with composing carbides, (See tab. 1.); the
percentage of carbide phase depends upon the Zr/C correlation:
(i) 50Zr : 50C –
ZrC0,9-0,95≈96,5±4% weight, cubic type lattice, В-1, period – 4,677х10-1
nm, crystallites size - 50-60х10-1 nm, Hv(0,2-1N)= 28-30 GPa.
(ii) 80Zr : 20C – ZrC
0,5-0,6 = 76,5±0,4 % weight + Zr-23,5±0,4 % weight, cubic type lattice,
В-1, period – 4,683х10-1 nm, crystalline blocks size - 120-180х10-1nm,
Hv(0,2-1N)= 8-10 GPа.
The results of
roentgenographic analysis of the phases are confirmed by the
investigation of energy spectrums of photoelectrons.
Besides, the increase
of the C content and the decrease of Me content brings about, together
with the MeC-phase, the appearance of a C-phase (diamond like) with
cubic type lattice, period – 3,567х10-1 nm, crystallites size ~ 500х10-1
nm and C-phase (graphite) of hexagonal type (2,511 and 8,241)х10-1 nm;
blocks size - 500х10-1 nm.
The level of
compressive stresses inside the plain carbide phases as well as
metallic and C phases is high approaching 0,2-0,56 GN/m2.
The typical
microstructure of surface and coating fracture of ZrC of 12-15 mkm
thickness on Cu is displayed on Fig. 5.
The ZrC deposition
occurs at the temperature range 150-200 оС forming coating with
nanocrystalline structure which withstand the temperature up to
900-1200оС, Fig 6.
The nanocrystalline
structure of coatings secures very high oxidation-and corrosion
resistant properties. The oxidation rate of nanocrystalline structure
coatings inside the pure oxygen current starts fast growing only from
750 оК.
In addition to high
oxidation-and corrosion resistant properties such coatings which are
formed by the joint sputtering of graphite and metal from the mosaic
targets give a very low coefficient of friction~ 0,06-0,15.
Resume
More than 10 years
intensive experience in the application of high rate magnetron
deposition systems to sputter a great variety of coatings types,
including coatings on nuclear fuel as well as in the investigation of
multi component coatings, i. a. different carbides clearly shows that
the HDRM technology cum mosaic targets is a sound alternative to the
chemical CVD technology to deposit multi film coatings on nuclear fuel
particles.
The joint sputtering of
graphite and metal is of various versions for the technology: for
example, both the sputtering of plain carbon and carbide films of
different structure & composition and mixed - Carbon-Carbide. The
technology which is ecologically safe and technologically universal
permits to have a very high deposition rate up to a few tens mkm/hour,
as a consequence, a high productivity.
Sources
-
Tchernikov A.S.,
Permiakov L.N., Kurbanov S.D., & others.
“Nuclear Fuel for HTGCR
based on the micro spheres of plutonium oxcide” – Atomic Energy, Vol.88,
#1, Junuary 2000, pp. 35-38.
-
Kazho Minato, Toru
Otawa, and Razuhiro Sawa & others– Irradiation Experiment on ZrC
Coated Fuel Particles for High Temperature Gas-Cooled Reactors –
Nuclear Technology, vol. 130, June 2000. pp 272-281.
-
T. Ogawa, K. Ikawa,
and K. Iwamoto “Chemical Vapor Deposition of ZrC Within a Spouted Bed
by Bromide Process” I Nuel. Mater, 97, 184 (1990)
-
Shiriajev
S.A.,Atamanov M.V., Mitin V.S. & others “The Production and Properties
of Composite Coatings of Metal-Carbon with nanocrystalline structure”
TPJ, 2002г., vol.72, pp. 99-104.
-
P.S. Kelly and R.D.
Arnell “Magnetron sputtering: a review of recent developments and
applications, Vacuum 2000, 56, p. 159.
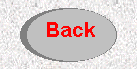